Capabilities
Capabilities
Probing and Mitigating Chemical, Electrochemical, and Photochemical Corrosion of Electrochemical and Photoelectrochemical Assemblies
Laboratory
Lawrence Berkeley National Laboratory (LBNL)Capability Expert
Francesca M. Toma, Nemanja Danilovic, Mike TuckerClass
CharacterizationSystem Integration
Node Readiness Category
2: High-Temperature Electrolysis (HTE)2: Low-Temperature Electrolysis (LTE)
1: Photoelectrochemical (PEC)
Description
This comprehensive node includes multiple experts alongside a suite of characterization and coating techniques for systematically assessing the chemical, electrochemical and photochemical stabilities of PEC, LTE and SOEC components and assemblies. In addition to characterization, this node includes mitigation strategies through application of functional or passivating coatings to provide corrosion protection to components and integration into assemblies for PEC, LTE and SOECs. Technology application area and readiness level are noted throughout.
Characterization
This suite of characterization techniques can be performed on various material, components and assemblies:
- High temperature chemical degradation [HTE 1]: Corrosion and oxidation of metals in the temperature range 500-1,000°C is a key phenomenon occurring during the operation of solid oxide and proton conducting electrolyzers and electrochemical hydrogen compressors. LBNL has capabilities for analyzing and mitigating high-temperature corrosion. Dedicated test stands allow oxidation of porous or dense specimens for hundreds or thousands of hours in controlled conditions in an environmental chamber (temperature, atmosphere composition, moisture content, PO2). Post-oxidation metallography, XRD, and high-resolution SEM and EDS allows quantification of surface scale and sub-scale formation, phase formation, elemental migration and depletion, and evolution of surface coatings.
- Molecular Foundry (http://foundry.lbl.gov) [LTE:1, PEC:1, HTE:1]: Is a worldwide accessible user facility for organic and inorganic synthesis but also world class characterization equipment including transmission electron microscopes through the National Center for Electron Microscopy (NCEM). EMN Node PIs and Project PIs have a unique opportunity to obtain express access using a Partner User proposal. The Partner Users would be able to use facilities on a limited basis to support a full Foundry proposal. Note that access is not guaranteed, and the timing and availability of Partner User and Full proposals should be negotiated with node PIs in consultation with Foundry staff to have the highest degree of success.
- Inductively coupled plasma mass spectrometry (ICP-MS) [LTE:2, PEC:1]: This technique can provide complimentary information on the chemical content of the electrolyte and of that one of the functional material, after its digestion. The sensitivity of this technique is in the part per trillion range. Leaching of elements from the (photo)electrochemical assemblies can be detected with high precision. We have a wide range of different standards.
- Electrochemical atomic force microscopy (EC-AFM) [LTE:2, PEC:1]: This technique enables the time-lapse topological observation of photoelectrocatalytic and electrocatalytic materials under in situ and operando conditions with very high (<10 nm) spatial resolution.
- X-ray fluorescence spectrometry (XRF) [HTE:1, LTE:1, PEC:1]: Allows for spatial elemental detection (greater than Na) on large samples up to 200mm x 60mm. With capability to resolve coating composition for up to 20 layers. Information on coating or catalyst layer uniformity, dissolution and migration from a catalyst layer or photoabsorber, quantifying and locating impurities and contamination in membranes.
- Low (25-90 °C) and high temperature (400-900 °C) electrochemical [HTE:1, LTE:1, PEC:1], and photoelectrochemical [PEC] measurements: These techniques allow for evaluation of materials performance and stability under operating conditions.
Mitigation
The suite of mitigation strategies that can be deposited onto catalyst (HTE, LTE, PEC), photovoltaic and photoabsorber (PEC), electrode support (HTE, LTE) are as follows:
- Spray pyrolysis [HTE:1, LTE:1, PEC:1, STCH:1]: Capability enables high quality films to be deposited on many types of substrates, producing materials combinations that are not commercially available. This tool is an Exacta fully integrated Pyrolysis Precision Programmable Coating System (model: W5226, vendor: Sono-Tek). It has dual ultrasonic nozzles that are mounted a XYZ-gantry to precisely control deposition of material. The system is enclosed and capable of synthesizing materials at a range of temperatures from room temperature to 600 °C. Recipes have been developed for transparent conducting oxides and metal oxide films. Deposition size up to 300mm x 300mm.
- Atomic layer deposition [HTE:2, LTE:1, PEC:1, STCH:2]: GEMSTAR-6 Atomic Layer Deposition tool with heat control (model: GEMSTAR-6, vendor: Arradiance) for depositing highly conformal thin films with precise control of composition and thickness at relatively low temperatures.
- Infiltration [HTE:1, LTE:2]: Deposition of coatings onto dense and into porous metal structures can greatly reduce the oxidation rate and vaporization of the alloy constituent elements, including Cr. For porous structures, conventional line-of-sight techniques are not applicable. LBNL uses infiltration of precursor solutions and ALD to deposit conformal coatings within the metal structure. A wide range of coating materials can be deposited and processed further in air or reducing atmosphere up to 1,500 °C.
- Corrosion barriers [PEC:1]: Various protection schemes have been developed that can be used to easily protect the underlying substrates for PEC assemblies. This includes a patented epoxy and grid system that is amenable to many different substrate materials.
Capability Bounds
Almost all the systems are compatible with different sample geometries and very user-friendly. Case-by-case evaluations are necessary to assess how to best perform the experiment.
Unique Aspects
The specific combination of all these characterization techniques and possible mitigation solutions offers a thorough and complete analysis of electrocatalytic and photoelectrocatalytic materials properties in their working environment.
Availability
The various capabilities are in use and supported by JCAP and other programs but can accommodate additional projects. Foundry access will be obtained through Partner User proposals as well as Proposals.
Benefit
Evaluating the stability of materials for photoelectrochemical water splitting requires testing under conditions that are relevant to practical devices. The results obtained from these characterization techniques can be used to guide extensions of theory to consider intermediate (photo)chemical states and may provide enhanced predictive power for evaluating and interpreting the stabilities of promising new semiconductors for solar fuels applications. In addition, improved understanding of mechanisms governing photochemical stability will guide development of experimental strategies for stabilizing chemically sensitive semiconductors. This methodology can be applied for rigorous investigation of the stabilities of new and emerging functional materials under operating conditions.
Images
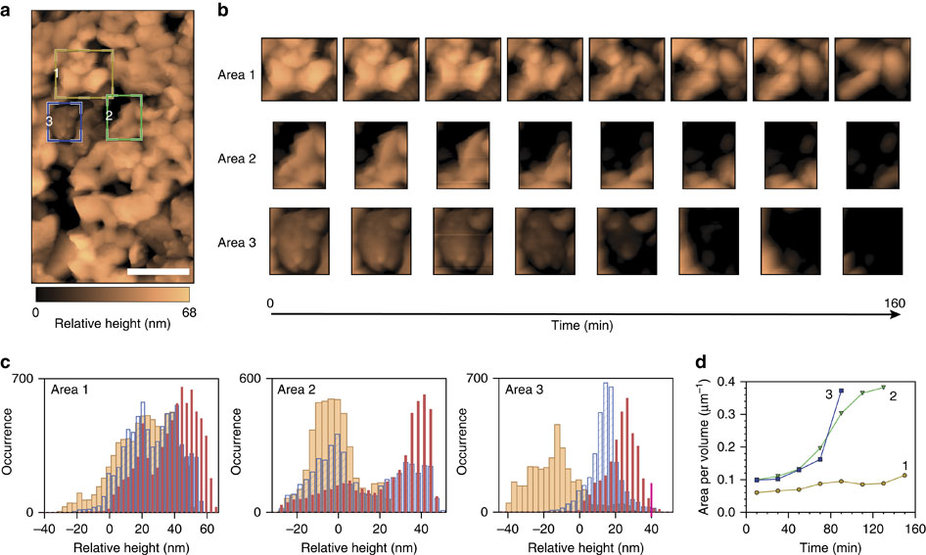
Figure 1. (a) EC-AFM scan (527 × 898 nm2; scale bar, 200 nm). The reported height is relative to a point on the underlying FTO substrate that is resolvable in all EC-AFM images. Common points have been used to align eight subsequent 1 × 1 μm2 scans of the same region, to correct for sample drift and rotation. Three coloured boxes indicate Regions 1 (yellow), 2 (green) and 3 (blue), whose temporal evolution were tracked in detail. (b) Aligned EC-AFM scans in the three regions indicated in a were used to monitor corrosion-induced changes to BiVO4 morphology at 20 min increments in 1 M KPi (pH 12.3). (c) Histograms showing height distributions in each of the three regions at the 10 (red)-, 70 (blue)- and 150 (orange)-min marks demonstrate that the heights of the BiVO4 film decreases over time. Height values <0 nm correspond to the underlying FTO substrate. (d) Progression of the surface area to volume ratio for region 1 (yellow), 2 (green) and 3 (blue) over the course of the 160-min test.

Figure 2. Environmental corrosion testing/characterization and its mitigation by thin film coatings.
References
J. Yang, J. K. Cooper, F. M. Toma, M. Favaro, J. W. Beeman, K. Walczak, C. Wang, C. Zhu, S. Gul, J. Yano, C. Kisielowski, A. Schwartzberg, I. D. Sharp, "A multi-functional biphasic water splitting catalyst tailored for integration with high performance semiconductor photoanodes", submitted.
F. M. Toma, J. K. Cooper, V. Kunzelmann, M. T. McDowell, Jie Yu, D. M. Larson N. J. Borys, C. Abelyan, J. W. Beeman, K. M. Yu, J. Yang, L. Chen, M. R. Shaner, J. Spurgeon, F. A. Houle, K. A. Persson, I. D. Sharp, "Mechanistic Insights into Chemical and Photochemical Transformations of Bismuth Vanadate Photoanodes", Nat. Commun. 2016, 7, article number: 12012.
L. Chen, J. Yang, S. Klaus, L. Lee, R. Woods-Robinson, J. Ma, J. Cooper, F. M. Toma, L.-W. Wang, I. D. Sharp, A. T. Bell, J. W. Ager, "P-type Transparent Conducting Oxide / n-type Semiconductor Heterojunctions for Efficient and Stable Solar Water Oxidation", J. Am. Chem. Soc. 2015, 137 (30), 9595-9603.
J. Yang, K. Walczak, E. Anzenberg, F. M. Toma, G. Yuan, J. Beeman, A. Schwartzberg, Y. Lin, M. Hettick, A. Javey, J. W. Ager, J. Yano, H. Frei, I. D. Sharp, "Efficient and Sustained Photoelectrochemical Water Oxidation by Cobalt Oxide/Silicon Photoanodes with Nanotextured Interfaces", J. Am. Chem. Soc. 2014, 136(17), 6191-6194.